How do Tesla coils work?
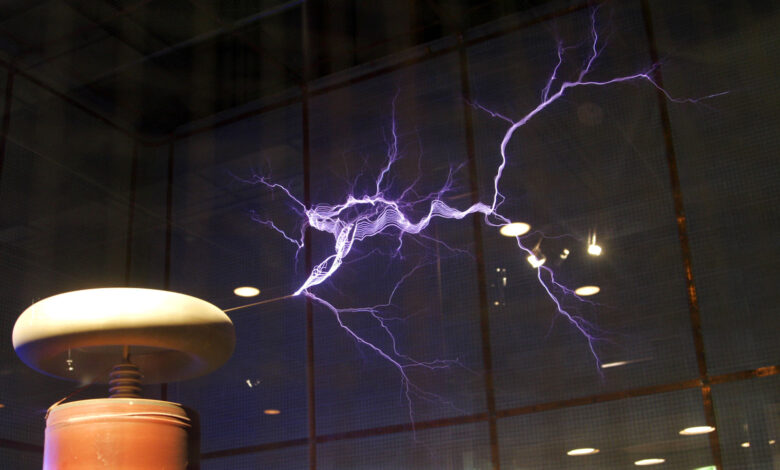
Nikola Tesla is probably one of the most appreciated scientists in the field of electronics because of all the inventions and technological advances that we use to this day. The two topics most influenced by him are the use of alternating current and wireless power transmission. It is precisely the latter that I want to talk to you about in this post, specifically through one of his most recognized inventions. In this post, we will see how do Tesla coils work.
Understanding the Logic Behind Tesla Coil Functionality
Before talking about how and why a Tesla coil is capable of generating those gigantic electric arcs, we need to understand Nikola Tesla’s objective and logic when developing this device. In the previous posts, we discussed how an inductor or coil works, explaining how an electric current flowing through a coil can generate a magnetic field and how the variation of a magnetic field can induce a current in another coil.
Understanding these two physical phenomena, it is easy to assume that we could apply current to a coil to generate a magnetic field and, in turn, use this magnetic field to induce a current in a nearby coil, thereby transmitting power wirelessly. The problem with this idea is that magnetic fields lose their strength quickly as we move away from the source. Consequently, as the two coils are separated, the energy transmission becomes increasingly inefficient.
Now, let’s see how the Tesla coil overcomes this limitation by taking this concept to the extreme. The logic is the same: to make an alternating current flow through a coil, but with extremely high voltage and a specific frequency, enabling energy transmission over greater distances. To achieve this goal, two main concepts must be understood.
The first concept is voltage increase through a transformer. In simple terms, a transformer consists of two coils: a primary winding, which is connected to a current source and generates a magnetic flux, and a secondary winding, which converts that magnetic flux back into a current. The interesting part is that if the number of turns on the secondary winding is greater than the number of turns in the primary winding, the output voltage will be larger than the input voltage.
A simple way to visualize this behavior is to imagine several batteries in series. Each time we add a new battery, the voltage adds up. If we make an analogy with each turn of the secondary coil, we can see that each loop acts as a voltage source, just like batteries. Therefore, each additional loop increases the total voltage.
Although we will discuss transformers in greater depth in the next video, one characteristic critical to the operation of Tesla coils is magnetic coupling. This refers to the efficiency with which the primary coil influences the secondary coil. For example, if an alternating current wave enters the primary coil, the wave coming out of the secondary coil will have the same shape but a different voltage.
In most cases, the goal is to maximize coupling efficiency, but not with Tesla coils. Their operation relies on a second concept: the use of a resonant circuit.
The Role of Resonant Circuits in Tesla Coils
A resonant circuit is made up of an inductor and a capacitor, which, as we have seen in previous episodes, are components capable of storing energy, though they do so in different ways—the capacitor with an electric field and the inductor with a magnetic field. The effect of connecting these two components in a closed circuit is that, in theory, we could keep an electric current flowing cyclically indefinitely.
Let’s suppose the capacitor is initially charged. Being in a closed circuit, it will start to discharge, creating a current that flows through the inductor and forms a magnetic field. Once the capacitor has fully discharged, the magnetic field will begin to lose its strength, inducing a current in the opposite direction and recharging the capacitor. This process continues until the inductor loses all its magnetic field, starting the cycle again.
Clearly, in the real world, this perpetual motion does not occur because the circuit itself has resistance, which gradually slows down the movement of electrons. However, this does not mean we cannot benefit from this behavior.
A resonant circuit has two major benefits. The first is that, although it cannot hold energy indefinitely, it can do so for a certain period, losing strength with each cycle. This is similar to a pendulum, which, with a single push, can oscillate at a regular frequency. Additionally, like a pendulum, if we periodically push it at the exact moment, we can amplify the end result even though we are only applying the same amount of force as initially.
The second benefit of resonant circuits is that they have their own natural frequency of oscillation, determined solely by the characteristics of their components—namely, the inductance of the inductor and the capacitance of the capacitor. Taking this into account, the inductor in the circuit generates an electromagnetic field whose oscillation matches this frequency, which remains constant.
Interestingly, another object behaves similarly: a tuning fork. When struck, a tuning fork vibrates at a specific frequency. In an experiment, if two tuning forks with the same vibration frequency are positioned near each other and only one is struck, the second will eventually start vibrating. This happens because the pressure changes traveling through the air from the first tuning fork move the second one at just the right moments to amplify its motion, much like the pendulum analogy.
Thanks to this behavior, if we position two resonant circuits and activate only the first one, the electromagnetic waves generated by its inductor will eventually induce and amplify a current in the inductor of the second resonant circuit. This enables wireless power transmission over much greater distances.
With all of this in mind, we can now return to the Tesla coil to understand how do Tesla coils work.
Understanding the Original Tesla Coil Model
Focusing on the Original Model
Nowadays, there are several ways to achieve the same or even better results, but we will focus on one of the original Tesla coil models due to its simplicity.
The first component we need is an alternating current source, which will connect to a common transformer. This transformer has a high magnetic coupling between its two coils, enabling us to obtain alternating current with a higher voltage but the same frequency as the initial source—typically 50 or 60 hertz.
The Resonant Circuit and Its Components
This new alternating current is then fed into a resonant circuit consisting of a capacitor and an inductor, commonly called the primary coil. This setup also includes an additional component that serves as a switch—essentially two metal pieces placed extremely close together without touching.
The purpose of this section of the Tesla coil is to make the primary coil emit an electromagnetic field at the resonant circuit’s frequency. As the transformer induces a current, charges accumulate in the capacitor, which has no other path for the current to flow. However, as the source voltage increases with each oscillation, the electrons seek an alternative path, eventually jumping between the metal pieces in the form of a spark. This spark quickly discharges the capacitor, but as soon as it dissipates, the capacitor once again becomes the most efficient path for the current, repeating the cycle.
Interestingly, the frequency of this cycle is not the same as the initial transformer’s frequency but rather the intrinsic frequency of the resonant circuit, determined by the capacitor’s charging and discharging behavior.
The Secondary Coil and Voltage Increase
Now, let’s focus again on the primary coil. It carries an extremely high voltage oscillating at the resonant circuit’s frequency. This generates an electromagnetic field oscillating at the same frequency, which the secondary coil can harness. The Tesla coil’s secondary coil, positioned near the primary, functions as part of a second transformer. The large difference in the number of turns between the primary and secondary coils significantly increases the voltage in the secondary coil.
This secondary coil is connected to the ground at one end and features a toroidal-shaped metal piece at the top. The toroid’s shape helps it accumulate a larger charge while preventing the corona effect, which would otherwise cause energy to escape into the surrounding environment. The corona effect, as we discussed in the episode on electrically flying spiders, occurs when there’s a significant potential difference between an object and its surroundings, especially at high, pointed areas like ship masts. The toroid’s design mitigates this effect, allowing charges to accumulate until the potential difference becomes so large that it ionizes the surrounding air, generating the iconic electric arcs of a Tesla coil.
Role of the Toroid and Charge Accumulation
But this isn’t all. As we noted earlier, resonant circuits have unique properties. The Tesla coil’s secondary coil and toroid form a new resonant circuit, though it lacks a physical capacitor. Instead, it relies on parasitic capacitance—a phenomenon that occurs whenever two conductors with different voltages are relatively close. With the Tesla coil’s high voltage, this effect is noticeable even across distances of several centimeters or meters.
To efficiently transfer energy between these two resonant circuits, they must share the same resonant frequency. While the frequency of the secondary circuit cannot be easily modified, adjustments can be made to the first circuit by varying its capacitance, ensuring both operate at the same frequency.
One final feature is critical to understanding how do Tesla coils work. The primary coil’s design intentionally reduces the magnetic coupling between the two coils. In practical terms, this means that when a voltage oscillation passes through the primary coil, the secondary coil generates a higher voltage due to the difference in the number of turns. However, the secondary coil will continue oscillating even after the primary coil stops. This behavior is analogous to a pendulum: the primary coil acts as the initial push, while the secondary coil keeps oscillating. This design allows the Tesla coil to generate extremely high voltages oscillating at the resonant frequency.
Wireless Power Transmission with Tesla Coils
Now that we understand how do Tesla coils work, let’s revisit its goal—transmitting power wirelessly. While the visible electrical discharges in the air are impressive, they serve no practical function and are, in fact, undesirable. By keeping the Tesla coil at a lower voltage to prevent these losses, we can generate only the electromagnetic field. For example, placing a fluorescent lamp near the coil causes it to light up. The electromagnetic field provides enough energy for the gas inside the lamp to emit ultraviolet light, which a phosphor coating converts into visible light. However, this use does not fully utilize the Tesla coil’s potential since it fails to take advantage of its resonant circuit properties. The range of such an application is also quite limited.
The true potential of the Tesla coil lies in pairing it with another resonant circuit operating at the same frequency. This configuration enables wireless power transmission over greater distances, similar to how a tuning fork can induce vibrations in another fork of the same frequency.
Finally, let’s address the million-dollar question: why don’t we use this technology for wireless power everywhere? The answer is that we already do—practically every day. Consider a radio signal: a large antenna emits electromagnetic waves at specific frequencies, and a radio receiver uses a resonant circuit tuned to receive the desired frequency. How these frequencies are used to transmit information is a topic for another post!
If you’re fascinated by the incredible science behind Tesla coils and want to bring this awe-inspiring technology into your own hands, check out the Musical Tesla Coils and Handheld Tesla Coils available at teslacoils.store. These innovative devices not only showcase mesmerizing electric arcs but also produce music by modulating the frequency of the sparks, creating a one-of-a-kind audio-visual experience. Perfect for STEM enthusiasts, educators, or anyone looking to own a piece of Tesla’s genius, these musical Tesla coils combine science, art, and entertainment in a truly electrifying way. Visit now and explore the possibilities of wireless power and sound like never before!
References
- Uth, Robert (December 12, 2000). “Tesla coil”. Tesla: Master of Lightning. PBS.org. Retrieved May 20, 2008.
- Eldridge, Stephen (January 7, 2024). “Tesla coil”. Encyclopaedia Britannica online. Encyclopaedia Britannica Inc. Retrieved February 10, 2024.
- Dommermuth-Costa, Carol (1994). Nikola Tesla: A Spark of Genius. Twenty-First Century Books. p. 75. ISBN978-0-8225-4920-8.
- Tilbury, Mitch (2007). The Ultimate Tesla Coil Design and Construction Guide. New York: McGraw-Hill Professional. p. 1. ISBN978-0-07-149737-4.
- Plesch, P. H. (2005). High Vacuum Techniques for Chemical Syntheses and Measurements. Cambridge University Press. p. 21. ISBN978-0-521-67547-5.
- Cvetić, Jovan M. (October 2016). “Tesla’s High Voltage and High Frequency Generators with Oscillatory Circuits”. Serbian Journal of Electrical Engineering. 13(3): 301–333. doi:2298/SJEE1603301C. S2CID 55561957.
- Haddad, A.; Warne, D.F. (2004). Advances in High Voltage Engineering. IET. p. 605. ISBN978-0852961582.
- Naidu, M. S.; Kamaraju, V. (2013). High Voltage Engineering. Tata McGraw-Hill Education. p. 167. ISBN978-1259062896.
- Sprott, Julien C. (2006). Physics Demonstrations: A Sourcebook for Teachers of Physics. Univ. of Wisconsin Press. pp. 192–195. ISBN978-0299215804.
- Anderson, Barton B. (November 24, 2000). “The Classic Tesla Coil: A dual-tuned resonant transformer”(PDF). Tesla Coils. Terry Blake, 3rd webpage. Archived(PDF) from the original on May 21, 2005. Retrieved July 26, 2015.
- Denicolai, Marco (May 30, 2001). Tesla Transformer for Experimentation and Research(PDF)(Thesis). Thesis for Licentiate Degree. Electrical and Communications Engineering Dept., Helsinki Univ. of Technology, Helsinki, Finland. pp. 2–6. Retrieved July 26, 2015.
- Denicolai, 2001, Tesla Transformer for Experimentation and Research, Ch.2, pp. 8–10
- Gerekos, Christopher (2012). The Tesla Coil(PDF)(Thesis). Thesis. Physics Dept., Université Libre de Bruxelles, Brussels, Belgium. pp. 20–22. Archived from the original (PDF) on October 1, 2015. Retrieved July 27, 2015., reprinted on The Zeus Tesla Coil, HazardousPhysics.com
- Gottlieb, Irving (1998). Practical Transformer Handbook: for Electronics, Radio and Communications Engineers. Newnes. pp. 103–114. ISBN978-0080514567.
- Burnett, Richie (2008). “Operation of the Tesla Coil”. Richie’s Tesla Coil Web Page. Richard Burnett private website. Retrieved July 24, 2015.
- Burnett, Richie (2008). “Coupling Coefficient”. Richie’s Tesla Coil Web Page. Richard Burnett private website. Retrieved April 4, 2017.
- Burnett, Richie (2008). “Tesla Coil Components, P. 2”. Richie’s Tesla Coil Web Page. Richard Burnett private website. Retrieved July 24, 2015.
In case you have found a mistake in the text, please send a message to the author by selecting the mistake and pressing Ctrl-Enter.
https://techplanet.today/storage/posts/2025/01/14/QawGGZReaJxOaPXPKTzNWY3Umrx2l5arQ0vmQRDW.jpg
2025-01-14 00:13:50